SMART Protocol Extends Silicon Qubit' Coherence by 100x
Still less computing time than an eye blink.
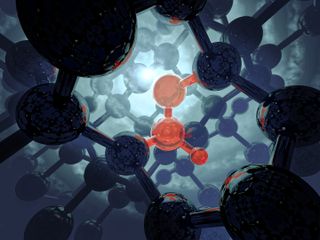
A team of researchers with the University of New South Wales (UNSW) in Sydney has achieved a breakthrough in spin qubit coherence times. The research took advantage of the team’s previous work on so-called “dressed” qubits - qubits constantly under the effect of an electromagnetic field shielding them from interference. In addition, the researchers leveraged a newly-designed protocol, SMART, which leverages the increased coherence times to allow individual qubits to be safely coaxed to perform the required computations.
The improvements allowed the researchers to register coherence times of up to two milliseconds - over a hundred times higher than similar control methods in the past, but still a ways from the amount of time your eyelids take to blink.
There are several ways of increasing the computing power available to a quantum system. Increasing the number of qubits - which can be thought of as akin to classical transistors - is one. Besides increasing the number of addressable qubits in a given system, it also matters whether the results these qubits provide are correct (for which several error-correction implementations are in development). Yet another way of improving performance is to increase the number of times qubits can hold their information before decoherence - the moment the qubits’ state collapses, leading to the loss of all information they contain.
In the case of spin qubits, whenever the electron stops spinning is the death knell for the qubits’ state. Ms. Ingvild Hansen, who handled the experimental work, was quick to point out the importance of the qubits' extended spin times. Se explained that “(...) we showed theoretically that we can improve the coherence time by continuously rotating the qubits. If you imagine a circus performer spinning plates, while they’re still spinning, the performance can continue. In the same way, if we continuously drive qubits, they can hold information for longer. We showed that such ‘dressed’ qubits had coherence times of more than 230 microseconds [230 millionths of a second].”
“Longer coherence time means you have more time over which your quantum information is stored – which is exactly what you need when doing quantum operations,” said Ph.D. student Amanda Seedhouse, whose work in theoretical quantum computing contributed to the achievement. “The coherence time is basically telling you how long you can do all of the operations in whatever algorithm or sequence you want to do before you’ve lost all the information in your qubits,” Amanda continued.
The researchers’ SMART (Sinusoidally Modulated, Always Rotating and Tailored) protocol aims to improve coherence times by reducing interference introduced into a qubits’ environment - while allowing for fine-grained control of each qubit.
One way of interacting with silicon spin qubits is to subject them to microwave fields, yet this has proven to be a taxing method: a microwave emitter has traditionally been required to control each of the working qubits. Yet sustaining so many microwave-based magnetic fields working in the quantum realm - alongside the scaling energy consumption and increased thermal dissipation from the slew of antennae - tends to increase the environmental noise. And higher environmental noise increases the chances that qubit decoherence occurs. Moreover, scientists’ attempts to increase control over qubit states worked against coherence times.
Stay On the Cutting Edge: Get the Tom's Hardware Newsletter
Get Tom's Hardware's best news and in-depth reviews, straight to your inbox.
All of this would be prohibitive against the requirements for full-scale quantum computing, expected to require millions of qubits harmoniously working towards a final computational goal.
Using a dielectric resonator, the researchers showed that the entirety of the qubit field could be controlled using a single antenna instead. The antenna, expected to handle millions of qubits simultaneously, works by sustaining the electron’s spin - the quantum property from which silicon qubits derive part of their charm. Another element is that silicon qubits could eventually leverage silicon manufacturers’ decades-long expertise in coaxing this material’s utmost performance and highest manufacturing yields.
But while it’s essential to sustain the entire qubit fields’ spin states (so that they don’t decohere), accurate calculations will still require that qubits are individually manipulated. For example, if changes in the microwave field affect all qubits similarly, then there wouldn’t be much to control what information each spin qubit represents.
The researchers devised and adopted the SMART protocol to interact more easily with qubit states. Through it, they could manipulate the spin qubits to rock back and forth instead of spinning in circles. Like the pendulum of a grandfather clock, each qubit was made to move back and forth. By interacting with the swing of each qubit through an electric field, the qubits were put out of resonance while maintaining their rhythm, allowing the researchers to get them to swing in different tempos compared to their neighbors (one “rising” as one was “falling”).
“Think of it like two kids on a swing who are pretty much going forward and backwards in sync,” says Ms. Seedhouse. “If we give one of them a push, we can get them reaching the end of their arc at opposite ends, so one can be a 0 when the other is now a 1.”
The UNSW researchers’ efforts have shown that groups of qubits can be controlled through a single, microwave-based magnetic source. In contrast, applying an electronically-controlled magnetic field can better control individual qubits. According to the researchers, the SMART protocol leverages a potential path for full-scale quantum computers.
“We have shown a simple and elegant way to control all qubits at once that also comes with a better performance,” says Dr. Henry Yang, one of the senior researchers on the team.
Francisco Pires is a freelance news writer for Tom's Hardware with a soft side for quantum computing.