Programmable DNA Offers Way to Accelerated General-Purpose Computing
Significant computing flexibility from the master of biological survival.
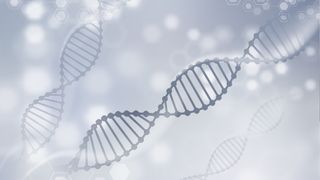
Chinese scientists across multiple national research institutions have published a paper on Nature that could spell a breakthrough in DNA-based computing. The researchers led by Lv Hui et al. published that they've designed a liquid, programmable-DNA-based computer that can process billions of different computing circuits - meaning that it can be used for general processing in a way similar to how CPUs are used today.
Due to the nature of its biological units, DNA computing offers the possibility of storing immense densities of data at up to 1 billion gigabytes per cubic millimeter - a boon for memory-heavy storage and processing tasks. With data densities like that, being able to manipulate DNA as an information processing system looks like something we'd want to pursue.
This is true especially when paired with the increased flexibility of a computing system that uses DNA strands as inputs and outputs. The computing we know is based on the ability to codify, store, and process information in a binary system: on or off, zero or one.
But DNA computing uses the four essential DNA molecules - adenine, thymine, guanine, and cytosine (ATGC), to build a computing system to store and process more information per operation. While transistors limit you to map either a zero or a one to it being powered up, DNA computing can encode 00, 01, 10, and 11 as A, T, G, and C. This allows DNA computing to offer more possible, condensed combinations of information that the binary system (0 and 1) can't easily reach.
Suppose we know that the four essential DNA molecules (A, T, G, and C) only bind in a particular manner between each other. We can use this property as a computing mechanism for additions and subtractions and more complex and broad (general) operations.
In DNA computing, adenine pairs with thymine, and guanine with cytosine; a short DNA strand made up of ATGC, for example, would only bind to TACG and ignore all other sequences. This predictability, this principle is the same one that allows Zelda: Tears of the Kingdom players to build calculators with in-game physics.
But it's one thing to be able to use DNA strands as a computing mechanism; it's another to make it so that you can control which strands connect as you begin scaling the computer with additional strands. Additional copies of each (and their additional information content) could connect haphazardly.
To introduce actual control into the equation, the research paper describes a DNA origami - a DNA sequence designed in such a way that it can become a 2D or 3D shape. Interestingly, this ability of shapes to provide different information elements is a principle that topological quantum computing and MC Esher's work explore and is fundamental in superconductivity, quantum mechanics, and others.
Stay on the Cutting Edge
Join the experts who read Tom's Hardware for the inside track on enthusiast PC tech news — and have for over 25 years. We'll send breaking news and in-depth reviews of CPUs, GPUs, AI, maker hardware and more straight to your inbox.
When applied to DNA origami structures, topology makes it so that it's more difficult for compatible DNA strands to adhere to each other. It's not enough that ATGC molecules find TACG molecules anymore - now, they have to be folded to fit together first. They become pieces in a computational puzzle.
Using this principle, the researchers used a DNA computer made of 30 logic gates (around 500 DNA strands), which they could use to compute accurate square root calculations. And in every field of computing and information processing, accuracy is king. With this proven accuracy, the researchers used their small DNA computer to identify three genetic molecules related to kidney cancer. Within two hours, the computer could correctly tell which samples possessed the identified molecules and which did not (within a pool of 23 total samples, 18 of which were healthy and 5 of which presented lung cancer disease).
Further gains in accurate, specialized computing forms such as quantum computing, DNA computing, and others will help researchers and humanity understand our world. To that end, the probability that the future computing landscape will look like the one from today. In particular, it would seem that different computing architectures will have to interoperate. Perhaps DNA computing will be most important in providing extremely-high-density cold storage, and not as a general processing computer floating inside a test tube (which it does; and I don't know about you, but I wasn't expecting that). Who knows?
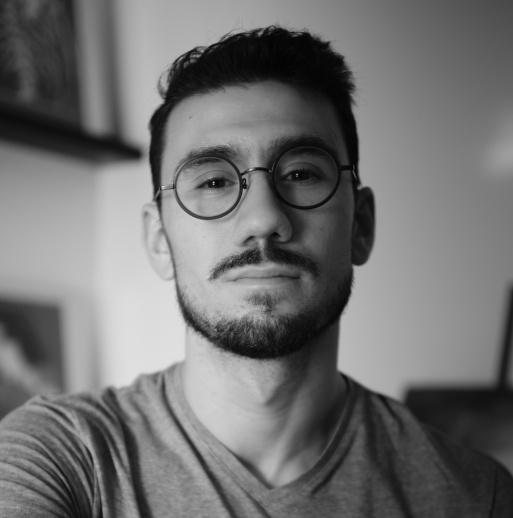
Francisco Pires is a freelance news writer for Tom's Hardware with a soft side for quantum computing.