New Quantum Magnet Promises Applications in Robotics, Electronics, and Sensors
Strain-tunable materials can bring forth chips that squeeze or bend.
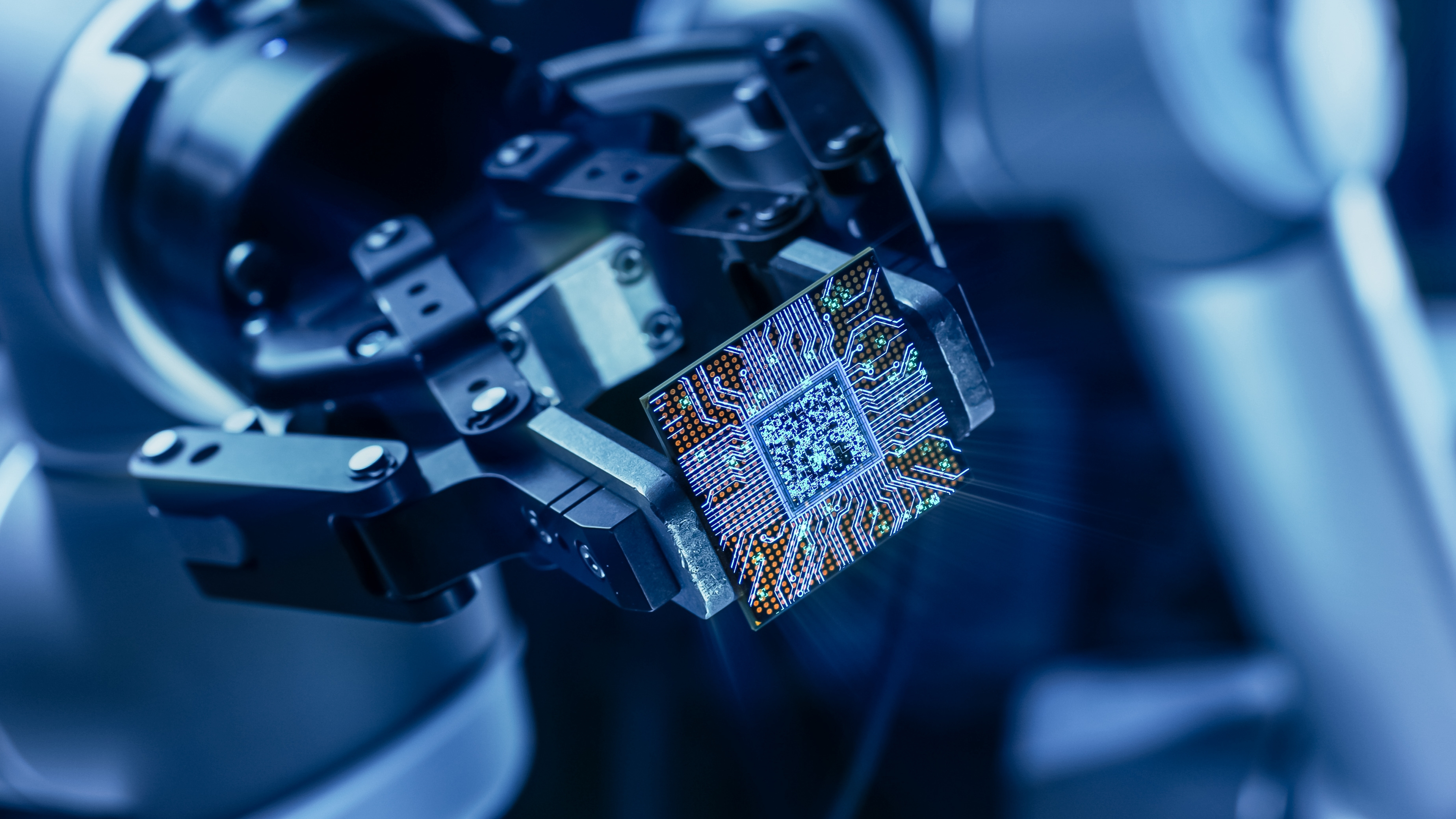
Researchers with the MIT Plasma Science and Fusion Center (PSFC) Hangu Chui et al have recently put a new spin on the well-understood technology behind magnets. While that may sound simplistic at first sight (how much better can a magnet become, after all?), the research unlocks new material applications. Magnets (and electromagnetism itself) being the basis of entire computational systems, improvements to base magnetic materials are expected to bring high-impact improvements to our handling of these fundamental forces.
Taking advantage of quantum effects, the researchers managed to control the anomalous Hall effect and Berry curvature, two fundamental physics barriers that stood against attempts of being put to work in a way that'd be useful for us. The research team's new paper, published in Nature, sheds some light on the usage of chromium telluride as a way to take advantage of both effects to both improve efficiency and performance. The impacted areas? Anywhere magnets matter: ranging through computation, electronics, and robotics.
The Hall Effect refers to a discovery made by 23-year-old Edwin Hall, back in 1879. Hall noticed that putting a magnet in a right angle against a vertical strip of metal with a current coursing through it deflected the current against the opposite end of the metal sheet (remember that electrical current is the ordered motion of free electrons).
This asymmetrical difference in current became known as the Hall effect. But with quantum mechanics, this asymmetrical behavior can be used to our advantage. Think of quantum mechanics as a way to look at what the Hall effect is actually doing at a particle-physics level, which, in turn, allows us to understand and affect the circumstances in which in manifests.
That's where the application of a quantum concept known as Berry curvature comes in: within quantum physics, it can be used to naturally deviate the flow of electrons (much like the Hall effect does). Except since it doesn't need the magnetic field, it's now known as the anomalous Hall effect, and it can be used to much more efficiently control the flow of electricity.
The researcher's work resulted in a material that displays this anomalous Hall effect even when squeezed and stretched - a hallmark for potential work in the field of flexible electronics. The material is built out of crystals: either aluminum oxide or strontium titanate base layers (half-millimeter-thick). Then, an atomic layer of chromium telluride, a magnetic compound, is applied on top of these layers. Due to the way it interacts with the base layers, the magnetic compound endows the crystal layers with flexibility.
But here, "flexible" means that when the material suffers a strain, it doesn't lose its ability to conduct electrons; they merely move through different pathways as allowed by the interaction between the anomalous Hall effect and the Berry curvature. This ability is why the researchers are calling this compound a "strain-tunable" material - because it naturally adjusts electric conductance according to the strain it's put under. Due to this, the researchers quote multiple applications in a number of highly-relevant fields.
Stay On the Cutting Edge: Get the Tom's Hardware Newsletter
Get Tom's Hardware's best news and in-depth reviews, straight to your inbox.
In robotics, strain-tunable materials can be used for "Soft sensors" - sensors that can stretch around existing biological elements (such as brain neurons in BCIs [Brain-computer Interfaces], for instance) in order to avoid damaging them or to better interact with them. Sensors that stretch according to environmental factors or bendable control mechanisms for artificial prosthesis are also opened up via this technology - not to mention the benefits for companies exploring such as Neuralink.
These strain-tunable materials also have applications in data storage - the stretchable material can store varying amounts of data according to how exactly it's stretched, which would bring definite benefits in density, and possible benefits in data retention.
Of course, any new technology depends on cost - cost of scaling being a limiting factor. How fast this technology is adopted depends on many factors, including the cost of the materials themselves, and how much work is needed to adapt currently-existing CMOS (Complementary metal–oxide–semiconductor) fabrication technology - the one that lives within our Best CPUs and GPUs of choice - to it.
But cost is met with investment, and for the looks of it, further work on these strain-tunable materials is to be done: this original study was partly supported by the U.S. Research Office, the U.S. National Science Foundation (NSF), the Massachusetts Institute of Technology, and a number of other U.S-based government and research bodies. It seems that momentum behind these might be a little bit more focused than usual.
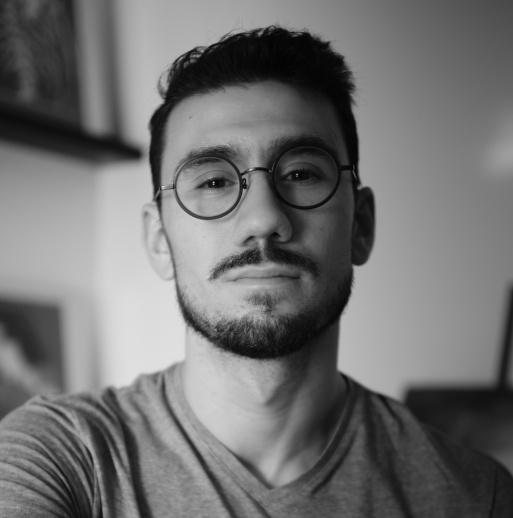
Francisco Pires is a freelance news writer for Tom's Hardware with a soft side for quantum computing.