How We Test Gaming Headsets
Introduction & Overview
What makes one headset perform better than another? What is legendary gaming sound, anyway? Purportedly, it was developed with professional gamers in mind, and every new headset supposedly comes with better, never-before-seen improvements that enhance your experience, particularly in first-person shooters.
What criteria should you use for comparing products in this category, and which buzzwords should you ignore? Before we start publishing our own reviews, we wanted to provide a scientific background to flavor the methodologies you'll see us use.
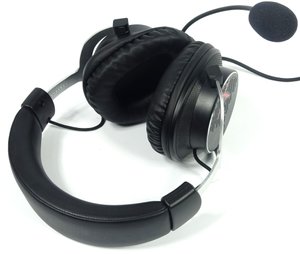
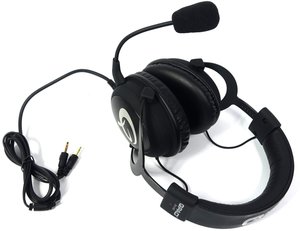
Today's story includes a deeper analysis of headsets and their performance. We'll cover the theoretical basics of sound, human hearing, and positional audio. You'll also come away with an understanding of what we measure and how. And in the end, digging into so-called gaming sounds yields some surprising results.
What Do We Have In Mind?
To evaluate whether a pair of headphones does provide the best acoustics and neutral sound representation, with the widest possible sound stage, it is necessary to examine the effects you hear in games, such as weapons, environmental noises, vehicle/aircraft sounds, and the quality of speech reproduction for different types of voices.
The gaming sounds we analyze come from titles like Battlefield 4, Far Cry 4, Fallout 3, and Crysis. They represent the "gaming soundscape" necessary to evaluate the quality of audio reproduction. Interestingly, many of the noises are cut off at around 16 kHz. And in some cases, games use some kind of clipping to make the sound seem even louder (compression).
Soon we'll see which frequency ranges are actually used in games and how certain sounds are reproduced. But first we need to cover a little bit of theory. After all, we don't want to nit-pick the headsets we review without telling you why we're being critical. It'll help for you to understand the method behind our madness.
A Bit About Sound And Waves
Sound waves are at the heart of our endeavor. We're looking at what happens between a sound source and the human ear as a receptor. Obviously, we want to create this sound using our test objects and hear it with our own ears. However, a lot can happen on the way between a source and its target (and also after the sound is physically recognized, as it's being transmitted, and when the brain interprets generated data).
Stay On the Cutting Edge: Get the Tom's Hardware Newsletter
Get Tom's Hardware's best news and in-depth reviews, straight to your inbox.
You may have already learned some of this in physics class, but sound is a purely mechanical oscillation process that, depending on the respective medium, has a specific speed of propagation (in air, it's approximately 330 to 340 m/s). The speed of sound is also influenced by factors like humidity and temperature, but not by air pressure. For the sake of simplicity, we'll ignore those factors here.
More pertinent to our discussion is the fact that sound travels through space in a wave-like manner. Depending on the pitch of a tone, the corresponding sound wave has a specific wavelength, which is easy to calculate; it's the speed of sound divided by the frequency. For example, a test signal of one kilohertz (that's 1000 oscillations/s) at a propagation (speed of sound) of 330 m/s gives us 330 meters divided by 1000. Thus, the wavelength would be 33 cm (0.33 m). At 100 Hz, the wavelength would be 3.30 meters. At 30 Hz, it's almost 10 meters!
How We Hear: The Eardrum
Sound reaches our ear and travels along the ear canal to the eardrum. Let's keep it simple and use our example test frequency of one kilohertz. This sinusoidal sound is nothing more than a specific kind of pressure wave hitting the eardrum. The pressure exerted on the eardrum changes periodically, following the respective frequency illustrated in the image above, since the sound only hits the eardrum from one side.
What we "hear" is the difference between the sound pressure and the constant ambient air pressure, which should be identical on both sides of the eardrum, canceling itself out. These differences are no larger than just a few millionths of a bar, while the average air pressure in normal weather at sea level is 1000 mbar. This also shows just how sensitive our hearing actually is.
Unfortunately, the ear is a little limited when it comes to perceptible frequency range. On average, we pick up somewhere between 16 Hz to 16 kHz or so. Depending on a person's age, this range may be a little narrower, especially when it comes to higher-pitched sounds.
Physiology Of The Ear
Imagine sitting in a concert hall with an orchestra playing. There, you hear the loudest parts at up to 100 phon (subjectively perceived volume). At the same time, a recording of the concert is made, and mixed to precisely match the sound as it was heard in the first row.
So impressed, you purchase this recording on CD. But when you play it back, you're disappointed that the sound played within the four walls of your home, as well as the sound conveyed through headphones, seems to be completely different from what you experienced at the concert. The reason for this is our ear, which strongly influences the subjective perception of volume according to frequency and actual sound level. The human ear is not a linear, let alone ideal transducer!
The frequency-dependent perceived loudness was analyzed in early 1933 by Harvey Fletcher and Wilden A. Munson, who, for the first time, translated the results into a comprehensive curve. It can be seen very clearly that, especially at low frequencies with decreasing sound level, the perceived volume is drastically reduced. Certain high-frequency sounds are affected by this natural characteristic of human hearing as well. As a result, various amplifiers and active speakers today provide some "hearing-corrected" setting or "physiological" volume correction (loudness function), even though such features should always be approached with a healthy level of skepticism.
The much-praised "linearity" throughout the sound spectrum can not be achieved without a very high sound level, since our own ear will always be the weakest link in the chain!
Another factor is the different degrees of attenuation for various frequencies in relation to the distance and angle to the sound source. The signals that reach our ears on the shortest and most direct route are called "direct sound." However, since sound propagates in a wave-like manner (similar to light), and sources don't emit sound in just one direction, so-called reflections occur. Sound hits the auricle and is reflected back (in a weakened state), similar to a ball on a billiard table.
For closed and semi-open systems, the space between the transducer and ear is in fact our audio sensor chamber, thus explaining how and why different materials and shapes can noticeably change and distort sound.
Sound Event And Auditory Event
Even if a specific sound source produces the same sound event (for example, a piece of music played in an endless loop), every human being will subjectively perceive the resulting sound in a completely different way.
In contrast to this so-called sound event, the subjective auditory event is individually defined by spatial and temporal characteristics. The relation between sound event (stimulus) and auditory event (sensation) is a complex matter, and it would be wrong to treat one exactly like the other.
Too complicated? Let's take a look at what these things mean in detail by comparing the real sound event (measurable values) to our subjectively perceived auditory event (subjective impression):
Sound event | Auditory event |
---|---|
Sound pressure level | Loudness (phon) |
Frequency | Melody (mel) |
Acoustic spectrum | Sound |
Position of sound source | Localization of the sound source and direction of the auditory event |
We already learned about sound pressure level, frequency, and the acoustic spectrum of complex sound events as a mixture of various frequencies. This leaves the position of the sound source and the impact that these four distinct properties ultimately have when they act in combination.
Frequency Ranges And Subjective Auditory Impression
By analyzing exactly which frequencies a device is able to reproduce, and how well or poorly this reproduction is, it is possible to evaluate, with a high level of certainty, the device's overall performance across the entire frequency spectrum.
It might help to check out the interactive representation over at independentrecording.net, which provides a good overview of individual frequency ranges, examples of important sounds found in that specific range, and their potential sources. Just click the static preview image or the text link!
This diagram shows that all frequency ranges from lowest bass to highest pitch are well occupied.
Meanwhile, the following table presents an overview of the most important frequency ranges we use to subjectively evaluate headsets and headphones.
Category | Frequency Range | Description |
---|---|---|
Lowest bass | 16 to 32 Hz | This area of the sub-bass octave is reached only by very few instruments, but may be essential for classical music. Only a handful of the speakers we test are able to reproduce this range to some extent, let alone in its entirety. |
Low bass | 32 to 64 Hz | The bass octave (32.7 to 65.4 Hz) contains many interesting instruments, as well as the effect track of clean-mixed Dolby soundscapes used in movies (so-called track 0) and certain game effects. It can include extremely low-tuned bass guitars, earthquakes, detonations, or big bass drums (kick drums) for those dance enthusiasts among us. Without low bass, everything sounds a little flat. |
Bass and upper bass | 64 to 150 Hz | Bass up to 150 Hz, which includes the great octave (65.4 to 130.8 Hz), contains the fundamental speech frequency of male voices and has a strong influence on the lifelike reproduction of male vocals. When we look at this range, that's exactly what we're looking for, along with the harmonization of different voices, including the localization of individual sources (choir). |
Lower mid-range | 150 to 400 Hz | Together with upper bass, the so-called fundamental range plays an important role in the subjectively perceived warmth and fullness of sound provided by a large number of instruments. The fundamental speech frequency of female voices can also be found in this area. We thus evaluate both individual female vocals and overlapping voices (choir) to assess the quality of spatial reproduction. |
Upper mid-range | 400 Hz to 2 kHz | The upper mid-range contains the 1 kHz point, which is still used as a reference for many measurements. Unfortunately, many cheaper devices are tuned with these frequencies in mind in order to optimize their specifications and impress potential customers. However, this range also plays a relevant role in good spatial resolution, especially when it comes to broadband noise. |
Lower treble | 2 kHz to 3.5 kHz | The human ear is most sensitive between 2 and 3.5 kHz, especially since the lower levels of this range are responsible for a good overtone reproduction of the human voice. These frequencies are also critical for the recognition of a voice or an instrument. Thus, in this context, this range relates to the respective tone color, or timbre. |
Medium treble | 3.5 kHz to 6 kHz | The success or failure of speech reproduction as a whole is determined by this range, since sibilant sounds (like the letter "s" and hiss sounds) fall into it. A good reproduction of these frequencies can make or break the brilliance of many string and wind instruments. When it's over-represented, this range may easily leave a metallic or scratchy impression. |
Upper treble | 6 kHz to 10 kHz | This range is important for a proper broadband representation of harmonics across many instruments and air sounds, as well as various percussion instruments. A popular subject to observe in this range is the frequently quoted jazz brush. While a guitar may suffer less from a bad representation, a violin might, in extreme cases, easily be degraded to a flute. |
Super high frequency | 10 kHz to 20 kHz | Only a few instruments are included in this range. However, for people with good hearing, it ultimately makes the difference between poor versus good reproduction. Anyone who claims they can hear higher frequencies than these is veering off into voodoo territory. They're the ones who'll spend big bucks on gilded speaker cables. After all, the actual ability to hear frequencies of ~8 kHz and up is heavily dependent on the listener's age. |
MORE: Best Deals
Current page: Introduction & Overview
Next Page Spatial Hearing, Surround Sound & A Lot Of Voodoo-
the nerd 389 In the article, you say that headphones always create the perception that the source is right at the head. This is incorrect. Newer techniques use either binaural recordings or HRTF approximations to reproduce entire auditory spaces.Reply
When employed correctly, these techniques allow for a more accurate reproduction of an environment than is possible with speakers.
If surround headphones employ those techniques, the result is true 3D sound (two angles, as well as distance). That said, the technology needed to pull this off in real time is still a few years away. For now, binaural recordings are the only way to achieve this effect in practice.
Finally, you should probably disclose the output impedance of your sources. Many headphones have drastically different frequency and phase response between low impedance and high impedance sources. The effect is most pronounced with low impedance headphones, like most gaming headsets (<64 ohms). Motherboards and sound cards usually have a high output impedance (10-100 ohms), while decent headphones amps have low impedance (<2 ohms). -
Spanky Deluxe I just don’t get Tom’s Hardware sometimes. They go out of their way to make top quality articles with great testing methodologies but at the same time make the website absolute garbage to use with half the stuff covered in abnoxious adverts, a terrible UI experience and then even more adverts for even worse stuff that is blatantly obviously scam sites like “How local <ISP LOCATION AREA> housewife earns $15962 per month from home” and the like. Sometimes adverts even stop you reading an article on mobile, i.e. actually block you pressing the next page button. I hardly ever read articles anymore here despite being a visitor for about 20 years as it’s just too much hard work to wade through the website design and adverts everywhere.Reply -
icycool_q1 Completely agree Spanky, I am coming here less and less because of the huge video playing throughout almost every article. In addition to that, I have very poor internet and it takes considerable time for this crap to even load. Hell, I'd be happy to pay them $5/year just to have NONE of this extraneous rubbish.Reply -
Co BIY Tom's reviews headsets?Reply
No category under peripherals ? and I don't remember a recent review. -
FormatC This is the intro review to understand, what we test and how. The headset reviews will follow soon. I'm testing headsets for the German site since a few years (my latest). I think, it is a good compromise for the masses to get more info and to understand, what we write. Not too flat like an unboxing video on YT and and not too in-dept like for the golden ears. ;)Reply
Finally, you should probably disclose the output impedance of your sources.
If measure with an good external amp, not on an onboard output. But with exception of a few pilot headsets I wasn't able to find a gaming headset above 32 ohms.
Newer techniques use either binaural recordings or HRTF approximations to reproduce entire auditory spaces.
As I wrote in the review, all this techniques are using your brain (and experience) to produce this immersion. But it doesn't help to make a bad headset better or to hear "more". ;)
-
hannibal I agree the article. I have always have good Hi-Fi speakers and headphones. Many years back I did Also buy Gaming headset (expensive one) and used it a while and was deeply dissapointed by the sound quality. Did go back to old music Hi-Fi headphones and was much more pleased and the next time I did upgrade my music system I did by the best Hi-Fi music headphones that I could afford at that moment and have been really pleased the sound quality.Reply
Good bass even in low volume, good balance at low volume, same when you increase the volume upp. So balanced and straight linear sound in all situations!
The multidriver system is interesting, but very difficult to make right. Have to check out some uber models From Senheiser and othe big audio manufacturers to see how well They can do it with their best systems. -
the nerd 389 @FormatCReply
I didn't mean to imply that you should measure with a low impedance source. Quite the contrary, actually. Most people don't have headphone amps, so it doesn't make sense to measure with one if that's your audience. All I meant was that the output impedance has a substantial impact on how headphones measure, so it's worth disclosing. It only takes a few minutes to measure if you have a decent multimeter and a couple of resistors lying around.
Your Creative unit should have an impedance between 10 and 50 ohms. That's a big enough range that it's a pretty notable amount of uncertainty in any measurements you do with it, though. It's worth mentioning that of the units I've measured, I've found almost no difference in output impedance between onboard audio and gaming-oriented sound cards. Even the external ones usually have at least 10 ohms of impedance.
Regarding the binaural and HRTF techniques, it's not really fair to say that they use the brain/experience to produce the effect. They simulate a physical phenomenon that occurs when sound hits the outer ear and head. You can even measure the effect (assuming you can sit still long enough).
The wording you chose is akin to saying "the lights in your room allow you to see using your brain/experience to allow you to perceive objects." Again, it's not technically incorrect, but it's not really going to help anyone understand it any better. Also, I wouldn't mention it except for the fact that the HRTF is likely going to become a major factor in mainstream audio in the next few years. It's already used in some high-end audio solutions, and it's expected to be a major ingredient in the next phase of VR. -
FormatC I'm building audio systems since 1979 and I followed in the past each trend to understand (or very often not), what the industry and their PR tried to sell us. I'm visiting a lot of meetings and workshops and it is every time very interesting to hear, what scientists, engineers and medicals said. Alone the inner structure of a human ear is so complex, that we can found thousands of variances of a different spatial immersion. For one person this fakes are working well, for others not. Or simply different. I also saw a lot of blind tests and the results were every time within the statistic. A good 50:50 for stereo vs. artificial sound manipulations.Reply
What I would say with this small story is:
Never believe, what the industry said. This is (mostly) pure PR and the utilization of the term Gaming, only to sell their low-end more expensive, is pure nonsense. Stickers and audio labels are nonsense too. Money makers. Good audio hardware can cost a lot of money but our job must it be in the first row, to find the the better pieces between all this crap. What we will do is a thing in the middle of this mostly senseless unboxing YT videos and the Hi-Fi magazines. Call it science for the masses. But we have in each case to take care, that we will stay understandable for all readers. Not so easy... ;)
BTW: The Creative amp is running between 10 ohms and 600 ohms not bad. But I'm trying each headset also onboard (if it is not USB) to see, how it performs. I'm worked together with MSI for example to improve their mainboard audio solutions and a lot of other companies have now a bigger focus at the audio part of their mainboard design (components, positioning). This was also a follow of my investigations of all this VGA-related influences ("you can hear what you see") and the mainboard layout. The fact, that you can see now on a lot of VGA cards low pass filters for all rails is a direct follow of this work. Together with improved PSUs... ;) -
JonDol 20524062 said:... it’s just too much hard work to wade through the website design and adverts everywhere.
There are already long years since AdBlockers became mandatory on THW in order to have a pleasant reading experience. Don't wait anymore before installing a few (not only one since some of them have agreements with ad networks and sneakily whitelist some ads to let them reach you). I personally have at least 3 AdBlockers on every web browser I use.
-
Virtual_Singularity 20524205 said:Completely agree Spanky, I am coming here less and less because of the huge video playing throughout almost every article. In addition to that, I have very poor internet and it takes considerable time for this crap to even load. Hell, I'd be happy to pay them $5/year just to have NONE of this extraneous rubbish.
Hmmm... Totally, agree. Save for the $5 a year part. =P
Edit: Good article all the same, as it's an area Tom's hasn't breached much, at least yet, as far as I've seen (or heard). Like the contributor with the outstanding DL/UL speed in the sig hints at: "not too flat like an unboxing video, and not too in depth like for the golden ears". I like that description. Though I personally wouldn't mind a "golden ears" type of review. But then, that'd likely remove or disqualify most gaming headsets from the topic altogether, along with readers? Though just so Tom's knows, some of us would indeed take the time to read the in-depth, "golden ears" stuff. I grew up in the analog world and usually still prefer it, maybe. Ha.