Materials Breakthroughs Paves Path to 2D Transistors
Technical breakthroughs will help deliver on the promises shown by 2D materials research.
Researchers have solved three fiendishly difficult technical challenges that were effectively blocking the realization of the potential shown by semiconducting 2D materials, a key ingredient to creating new atom-thick transistors that can reset Moore's Law. Thanks to the works of a multi-institutional team of researchers the production of high-quality 2D materials at a commercial scale now appears to be solved.
The advance of semiconductor development is threatened by natural restrictions imposed by the way transistors are fabricated and the materials that are used. This barrier to Moore’s Law has long been looming on the horizon, and forward-thinking scientists have been researching and developing alternative routes to deliver the continuous improvement that is sought.
One of the most likely practical ways that the semiconductor industry can put a new spring in its step is to replace silicon with so-called 2D materials to creat 2D transistors. Scientists looking closely at 2D materials have highlighted several attractive qualities which should help deliver significant improvements to performance, efficiency, and scalability. Intel’s Components Research (CR) Group, for example recently presented nine research papers, with some of them touting the use of new 2D materials as a route to developing processors with over a trillion transistors, by 2030.
Now three critical challenges to the commercialization of 2D materials have been solved, claim the international group of scientists, making the manufacture of 2D materials in single-crystalline form on silicon wafers possible. These challenges were specifically described as follows:
- precise kinetic control of layer-by-layer 2D material growth,
- maintaining a single domain during the growth for uniform thickness, and
- wafer-scale controllability of layer numbers and crystallinity.
You can read the full paper for further details about each of these challenges and how they have been solved by the processes invented by the multi-institutional team. The work is detailed in a paper titled ‘Non-epitaxial single-crystal 2D material growth by geometric confinement’ and published by Nature magazine.
Sang-Hoon Bae, one of the project leaders, and a professor of mechanical engineering and materials science at the McKelvey School of Engineering at Washington University in St. Louis, certainly seems confident in the impact of the research. “We believe that our confined growth technique can bring all the great findings in physics of 2D materials to the level of commercialization by allowing the construction of single domain layer-by-layer heterojunctions at the wafer-scale,” explained Bae. “Our achievement will lay a strong foundation for 2D materials to fit into industrial settings.”
As with all research of this nature, it could be years before we see 2D materials used in practical applications. However, with companies like Intel and Samsung deeply involved in this project — and the fact that Intel already has 2D Gate All Around (GAA) transistors in its research pipeline — that future might come sooner than you think.
Stay On the Cutting Edge: Get the Tom's Hardware Newsletter
Get Tom's Hardware's best news and in-depth reviews, straight to your inbox.
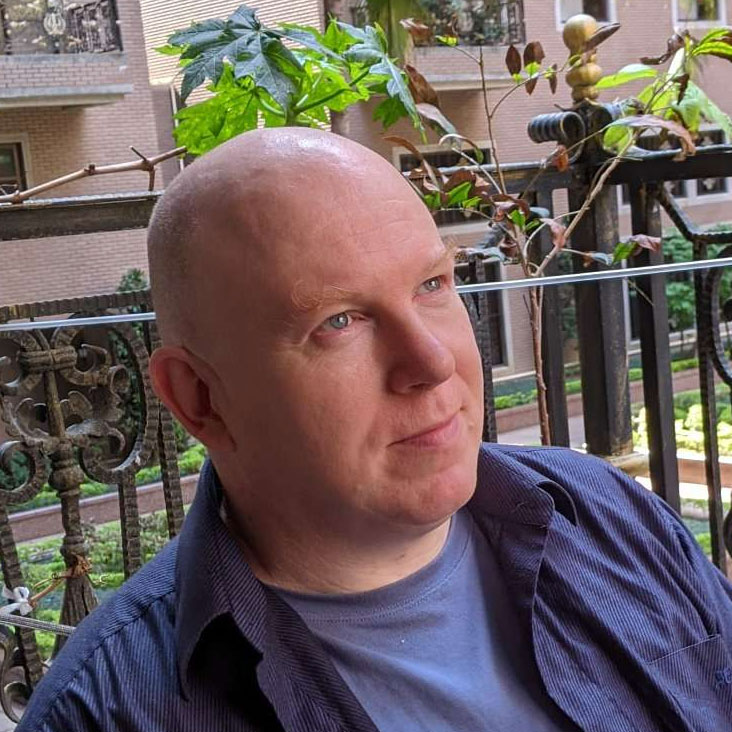
Mark Tyson is a news editor at Tom's Hardware. He enjoys covering the full breadth of PC tech; from business and semiconductor design to products approaching the edge of reason.
-
hotaru251 someone call Jensen...he might want to get a diaper ready when moores law isnt "ended" as he claims as excuse to keep GPU prices increasing.Reply -
Eximo Well, give it ten to twenty years when production scaling is achieved...Reply
If you think a CPU with two or three layers of stacking is expensive, wait until you have hundreds of layers. -
helper800 Just wait until we have the materials science to increase the efficiency of power transfer. All the cooling we currently need for all electronics is because of the small amount of resistance to the transfer of power. When we either create or find a way to reduce this resistance, without exotic means, we could essentially put as much power as we want into any part with very little cooling required. Imagine how small our electronics could be if cooling systems only needed to be 1/10th the size they are now.Reply -
TJ Hooker
What "transfer of power" are you referring to?helper800 said:Just wait until we have the materials science to increase the efficiency of power transfer. All the cooling we currently need for all electronics is because of the small amount of resistance to the transfer of power. When we either create or find a way to reduce this resistance, without exotic means, we could essentially put as much power as we want into any part with very little cooling required. Imagine how small our electronics could be if cooling systems only needed to be 1/10th the size they are now. -
Eximo Likely speaking of room temperature superconductors and the like. Not likely to happen anytime soon. Power requirements will go down in 2D materials simply because there is less mass doing the logic.Reply
There have been efforts to integrate cooling solutions into silicon and die stacks, even delivering power via liquid coolant. That hasn't really made it that far. -
helper800
From the PSU through circuitry and board components to CPU and other major parts.TJ Hooker said:What "transfer of power" are you referring to? -
helper800
The the amount of power lost as heat to components and everything in-between is what I am talking about. If we can reduce this to less than a few percent, it would revolutionize electronics.Eximo said:Likely speaking of room temperature superconductors and the like. Not likely to happen anytime soon. Power requirements will go down in 2D materials simply because there is less mass doing the logic.
There have been efforts to integrate cooling solutions into silicon and die stacks, even delivering power via liquid coolant. That hasn't really made it that far. -
TJ Hooker
Most of the power is consumed (i.e. converted to heat) by the chips themselves. Not as losses between the chips and the power source. Even if you eliminated all losses between the PSU and the actual silicon dies, it wouldn't make a big difference in cooling requirements, because most (active) cooling is already basically just for the heat given off by the dies themselves. I guess you could get rid of VRM heatsinks though.helper800 said:From the PSU through circuitry and board components to CPU and other major parts. -
helper800
The power lost as heat no matter where it occurs is due to materials inefficiency. All power lost as heat is due to resistance.TJ Hooker said:Most of the power is consumed (i.e. converted to heat) by the chips themselves. Not as losses between the chips and the power source. Even if you eliminated all losses between the PSU and the actual silicon dies, it wouldn't make a big difference in cooling requirements, because most (active) cooling is already basically just for the heat given off by the dies themselves. I guess you could get rid of VRM heatsinks though. -
bit_user
As long as semiconductors are capable of reducing entropy, they'll be consuming energy.helper800 said:The power lost as heat no matter where it occurs is due to materials inefficiency. All power lost as heat is due to resistance.
Do you have any figures on how much power is lost through distribution (i.e. copper traces) vs. transistors and other components?