Superconductor Levitates At Room Temperature, But Questions Remain (Updated)
Two different universities have shown LK-99 floating in the air.
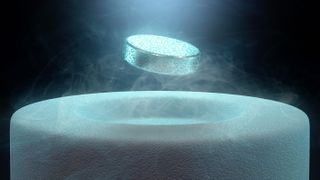
The worldwide scientific community is still working around the clock to verify claims of a new superconductor that might yet revolutionize human civilization. Now, we have two pretty convincing videos showing the substance floating in the air. Scientists with the Huazhong University of Science and Technology claim to have replicated LK-99's levitation abilities at room temperature, which they showcased in a video uploaded to Bilibili. And Zhang Chiang, from Wuhan University also in China, has uploaded a new video as of Saturday.
This is an encouraging sign: one of superconductivity's hallmarks, magnetism due to the Meissner effect, seems to be a replicable feature of the copper-lead-apatite compound. If only it were "that easy" to confirm (and understand) the material's zero electric resistance capability and how it manifests.
⋇ Beijing LK-99 Levitation Video Author Admits Fraud, Takes it Down
⋇ 16 Teams Race to Validate Superconductor Breakthrough, Find Mixed Results
⋇ Scramble to Validate Superconductor Breakthrough Confirms Zero Resistance, With a Catch
⋇ Superconductor Breakthrough Findings Replicated, Twice, in Preliminary Testing
The first video below comes from the scientists at the Huazhong University of Science and Technology and, though it's hard to see, there's a small black substance that's allegedly LK-99.
The second video, uploaded to Billibilli by Zhang Chiang, an assistant engineer and doctoral student at the Department of Metallurgical Engineering and Materials of Wuhan University of Science and Technology, showcases what’s claimed to be an LK-99 flake showcasing one crucial aspect of a superconductor’s levitation: flux pinning.
Huazhong University Video
Wuhan University Video
Flux Pinning
The Wuhan University video showcases an LK-99 flake levitating above a powerful magnet. But levitation itself isn’t a sure-sign of a superconductor: most metallic elements showcase diamagnetism by themselves. What differentiates a superconductor’s levitation is that it exhibits flux pinning - an emergent levitation capability that occurs as the pre-existing magnetic field (from the lower magnet) interacts with the superconductor.
The way to differentiate between diamagnetic and flux-pin levitation is that in “normal” levitation, the floating object can be disturbed by outside forces, including gravity - this leads to a floating rock that just can’t seem to stand still, as it glides, balances and wobbles above an ever-changing tug-of-war between it, the magnetic field, and external forces (such as a gust of air, for instance).
Flux pinning, however, pins the magnetic field lines within the superconductor itself. Type-II superconductors (such as LK-99 appears to be) feature internal magnetic vortices; flux pinning happens when the bottom magnet’s own magnetic field (which is actually quantized, that is, discretely composed of impossibly thin action lines, and not a formless cloud) interacts with these magnetic vortices and pinning centers are created.
Stay On the Cutting Edge: Get the Tom's Hardware Newsletter
Get Tom's Hardware's best news and in-depth reviews, straight to your inbox.
As the word implies, these centers “pin” the interaction between forces, locking the levitating superconductor in place. In the Wuhan University video, this is seen as the LK-99 flake stays pretty much locked in position above the magnet, despite being poked with the external, probing force of a simple pen
Where LK-99 Tests Stand
The clear-cut update is this: despite the fact the researchers could replicate LK-99's levitation at room temperature, there's still no successful replication of the announced room-temperature LK-99 superconductivity. For that to happen, both the Meissner-effect magnetic field and zero electric resistance are required from the same sample. And while scientists have previously shown that LK-99 does have zero resistivity at -163C, they haven't yet proven it has those properties at room temperature.
So what we're left with (still) is several failed or partially failed replications - and a whole world of additional knowledge on LK-99. The Wikipedia live tracker is one of the best places for anyone looking for up-to-date information on the (public) replication processes currently underway.
The replication difficulties and the nebulous history around superconductors (which have seen several similar room-temperature superconductor claims announced, published, and retracted) combine into a visible, waving, giant red flag. So remember to remove your rose-colored glasses. LK-99 is a fickle thing, and the ground it's standing on is filled with question-shaped potholes.
As our understanding of LK-99 improves, the fuzzy road ahead become slightly clearer. Unfortunately, it seems that the material's characteristics themselves may both be its boon and its blight. That's not to mention the fact that the original scientists did a shoddy job of documenting how they created the material, leaving scientists to patch together samples with a somewhat incomplete cookbook.
As we've explored before, LK-99 is a compound made from reacting lead sulfate with a copper-phosphorous compound. The process through which this compound becomes LK-99 requires that the materials be baked at high temperatures for around 24 hours in a vacuum. This is slightly easier to achieve than it sounds, as several Twitter/X posts and videos of people "owning their own LK-99" will show you (there's also the eternal memory of a Russian soil scientist and her kitchen counter as the first claimed independent synthetization of LK-99).
And adding great to good, the materials aren't even expensive to procure — the materials are all relatively cheap and abundant. But the biggest problem with LK-99 doesn't seem to involve its synthetization; the problem is the lack of control over the chemistry and quantum processes that occur during the fabrication process itself.
Crystals, it turns out, are fickle things. And the way LK-99 seemingly becomes a superconductor has to do with how many lead particles are replaced by copper. As it stands, it seems that the more copper that replaces lead in the final mixture, the purer the resulting compound is (which translates into it showcasing both the emergent levitation courtesy of the Meissner and zero resistance to electrical conductivity).
But that is both the solution and the problem; for now, there's no way for researchers to know what the synthetization process will actually do at an atomic level. So the scenario we're arriving at is that sometimes, there may simply not be enough superconducting elements in a given LK-99 batch for it to showcase any of the superconductive properties we're all hoping it does. It's actually in the formula: the "x" values in Pb10-xCux(PO4)6O, as it's represented in chemistry parlance, mean that it's uncertain just how many of the 10 base lead atoms are replaced with copper atoms. But it seems that the higher the number, the better.
To complicate things even further, however, it's not just a case of having as many copper atoms replace lead as possible; the places where these substitutions occur in the crystal also matter. It seems that some locations are better for unlocking LK-99's superconducting capabilities than others, and for now, once again, we have no way to "pick and choose" what happens during the synthetization process.
Adding insult to injury, the same LK-99 batch can have different ratios of copper atoms replacing lead across its volume. Some will be high, which is good for levitation and bringing a twinkle of excitement to our eyes; some will be low, resulting in a mostly inert compound that would be better used as a doorstop.
And that's saying nothing about how even the most random and seemingly insignificant variances in any of the synthetization steps can introduce unknown variables into replication attempts themselves — especially when researchers are following already badly-documented processes.
Cue the number of failed replication attempts, which, if all this pans out, is likely to keep increasing until it comes to a point where we can design a new synthetization process that improves yield. With all these moving parts, however, it's no wonder we're still walking across a dark room.
It’s still likely that the LK-99 saga will end in disappointment, despite all the lessons and science data being collected and worked on as a result of this. Perhaps it’ll truly happen as some have said (and we ourselves did), in that this discovery will follow the path of cold-fusion. Even if it does, all lessons learned here will inform our future - and the upside is simply too great for us not to try.
Update (Aug 5th): We've added coverage of the second video from Wuhan University.
Francisco Pires is a freelance news writer for Tom's Hardware with a soft side for quantum computing.
-
Kridian Superconductor Levitates At Room Temperature, But Questions RemainNo-no, that's good for me. Carry on.Reply -
Co BIY Anyone found any examples of basic journalism on the subject ?Reply
Like someone going and actually talking to the original researchers and asking them about their groundbreaking work? and why it was published in such an unorthodox manner.
We have their names and know their University affiliation.
I understand it might not be quite as cool as watching science tick tocks. But possibly informative. -
PlaneInTheSky I think the "superconductor could limit a power grid's losses" argument is silly.Reply
We currently lose far more than that 10% power loss from resistance, due to simple overcapacity from intermittent energy from wind and solar.
If governments cared about grid losses we would be turning that energy into hydrogen instead of shutting off wind turbines. -
OriginFree PlaneInTheSky said:I think the "superconductor could limit a power grid's losses" argument is silly.
We currently lose far more than that 10% power loss from resistance, due to simple overcapacity from intermittent energy from wind and solar.
If governments cared about grid losses we would be turning that energy into hydrogen instead of shutting off wind turbines.
Or for those of us on the "ring of fire" geothermal power.
That said, with the eventual push for electric vehicles (or at least plug in hybrid) + at home / in building power storage (think Tesla Powerwall) overcapacity loss will probably become a thing of the past.
That's not even accounting for the increased demand for heating / cooling as this wonderful climate change keeps giving us more extreme weather. -
kjfatl
We presently have high current DC power lines that arc cryogenically cooled in order to reduce overall system cost. We aren't looking for one silver bullet that solves all of our issues. It might turn out that this discovery is totally useless for power transmission, but it might also result in frictionless travel on interstate highways facilitated by magnetic bearings. It could be used in an unexpected way by increasing the efficiency of internal combustion engines, giving us a Chevy Tahoe that geta 100mpg using a gasoline engine.PlaneInTheSky said:I think the "superconductor could limit a power grid's losses" argument is silly.
We currently lose far more than that 10% power loss from resistance, due to simple overcapacity from intermittent energy from wind and solar.
If governments cared about grid losses we would be turning that energy into hydrogen instead of shutting off wind turbines. -
derekullo
The probably with hydrogen is that it is very hard to store being such a small molecule.PlaneInTheSky said:I think the "superconductor could limit a power grid's losses" argument is silly.
We currently lose far more than that 10% power loss from resistance, due to simple overcapacity from intermittent energy from wind and solar.
If governments cared about grid losses we would be turning that energy into hydrogen instead of shutting off wind turbines.
If you fill up your car with gasoline you can be confident that if you come back in a week youll have very close to a full tank.
With hydrogen that won't be the case.
vJjKwSF9gT8:647View: https://youtu.be/vJjKwSF9gT8?t=647 -
PlaneInTheSky
Hydrogen + carbon monoxide = liquid synthetic fuel you can just as easily store and transport as diesel or gasoline. And it's CO2 neutral.derekullo said:The probably with hydrogen is that it is very hard to store being such a small molecule.
If you fill up your car with gasoline you can be confident that if you come back in a week youll have very close to a full tank.
With hydrogen that won't be the case.
Hydrogen + nitrogen gas = ammonia which is easy and safe to store and transport.
Sure, that extra step to store hydrogen in a different carrier costs more energy, but currently overcapacity from intermittent energy, especially offshore wind that can generate a lot of energy on windy days, is literally going to waste.
European countries are starting to build islands next to offshore wind to store hydrogen though, so the energy stops going to waste.
-
Friesiansam If this is genuine, better known, respected institutions will replicate it. Then, in time, finds ways to make use of it. However, I am also sceptical.Reply -
oofdragon Would not be surprised if it's legit, this "race" started as soon as USA made the aliens disclosureReply