Overclocking Core i7-3770K: Learning To Live With Compromise
We recently took our first look at Intel's Ivy Bridge architecture. Then, we evaluated its efficiency. Now, we turn to overclocking. Recently, each of Intel's die shrinks has helped increase frequency headroom. This time, however, we ran into some walls.
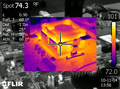
Ivy Bridge Overclocking: What Does It Entail?
Lower power consumption, purportedly less heat dissipation, a smaller die size, lower manufacturing costs for Intel...but does the 22 nm Ivy Bridge design also leave less room for mainstream overclocking? Our launch coverage (Intel Core i7-3770K Review: A Small Step Up For Ivy Bridge) revealed that overclocking the new processor design wasn't any more fruitful than the already-mature 32 nm Sandy Bridge-based Core i7-2700K flagship. Although stock temperatures were lower, they ramped up very quickly once we started applying the voltages thought necessary to approach 5 GHz on air.
Overclocking: What’s Required?
The length of time a transistor in a digital circuit delays an electronic signal depends on its size, fabrication technology, layout, temperature, and operating voltage. The highest achievable clock rate of a circuit depends on this delay and the number of logic levels that a signal has to traverse in a single clock period. The latter number is fixed (and dependent on the processor's architecture). So, for overclocking, we focus on how a transistor's latency is affected by its supply voltage. A higher supply voltage can shorten the delay, but will also raise the transistor’s power consumption. Cranking up the clock frequency also increases the dynamic power draw per time unit, and thus further raise the circuit’s power consumption, leading to a hotter chip.
Both effects, taken together, explain why overclocking at an elevated CPU voltage increases the power draw and heat output, and why cooling an overclocked CPU can quickly become challenging. As in sports or any engineering discipline, trying to eke out that last couple of percentage points is most difficult.
CPU manufacturers have put in some safeguards against reckless overocking by inexperienced users (and unscrupulous system builders); starting a few years ago, both AMD and Intel started shipping most of their models with locked multipliers, releasing more advanced models opened up with overclocking in mind. Of course, enthusiasts know that they can either tweak the multiplier through their BIOS or through a Windows-based utility provided by many vendors for easier access to those settings.
In case of Intel's unlocked Ivy Bridge-based K-series SKUs, the highest CPU multiplier was increased to 63x (from Sandy Bridge's 57x ceiling), translating to a theoretical 6.3 GHz limit if you don't touch the 100 MHz BCLK. Going higher requires changing the base clock, which is rather difficult. Above a 110 MHz threshold, very few systems are stable. Be that as it may, it's going to take more than conventional cooling to hit those clock rates. In reality, you'll only see the limits of these architectures pushed in overclocking contests and YouTube videos.
Overclocking: Expectations
Stay On the Cutting Edge: Get the Tom's Hardware Newsletter
Join the experts who read Tom's Hardware for the inside track on enthusiast PC tech news — and have for over 25 years. We'll send breaking news and in-depth reviews of CPUs, GPUs, AI, maker hardware and more straight to your inbox.
In the past, shrinking gate lengths have been seen to increase overclocking headroom. Smaller transistors require less voltage and consume less power, generally leading to better overclocking margins. Intel's Sandy Bridge-based K-series models easily achieved 4.3 to 4.6 GHz using air coolers, sometimes scaling even higher. Thus, our expectation for Ivy Bridge (along with many other enthusiasts, we'd say), was closer to 5 GHz.
However, we failed to achieve that goal, despite multiple tests in multiple countries using multiple Ivy Bridge-based samples. But we also received reports that Intel's 22 nm chips can break through speed records if you overcome their rapid heat-ramp using extreme measures like liquid nitrogen.
Knowing that LN2 is impractical in a production environment, we set out to achieve the highest overclock possible using conventional air cooling, discussing the causes of Ivy Bridge's limitations along the way.
Current page: Ivy Bridge Overclocking: What Does It Entail?
Next Page Overclocking Ivy Bridge: Treating This Hot-Head Gingerly-
mayankleoboy1 Nice review!Reply
1. Are there plans to release any K CPU's without the HD4000? will they OC higher?
2. Any chance of intel releasing a second stepping of K-series IB chips? -
mayankleoboy1 i agree that SB chips spoiled the OC world by making OC so easy and fruitful. IB has nothing to compare it to except SB.Reply -
vilenjan Good old Intel. No competition and look what happens, the new generation is barely an upgrade over the previous. Anyone remember Intel PII 450s and the PIII 450s?Reply -
ta152h I'm not sure why the reviewers spent so much time figuring out the shrink made the core more dense, and somehow thought this was significant. I mean, really, this happens virtually every shrink, and this time the power dropped more than normal. Common sense should be telling people this is not the cause, but somehow people aren't understanding this.Reply
It's very interesting that replacing the paste makes so much difference. This is obviously something Intel is aware of, since they do plenty of testing, and obviously chose anyway. Would a few pennies be worth it for a processor that is clearly on the higher end of the scale? Probably not.
Most likely, they want to keep selling their real high end processors, and it just won't do to have the 3770K beating their 2011 processors, or being very competitive with the successors to that line when they come out. It makes perfect sense. The 3770K is still a great processor, but if you're really looking for the best, it simply will not do. You're forced to buy the more expensive 3960X, and later the even better IB successor to it, which you can bet will have far better paste, and so will overclock significantly better.
It's genius. A great product for the vast majority, while leaving more expensive products as the best option for that elite that will actually spend $600 to $1000 for a processor.
Well done, Intel. It's not like AMD has anything to say about it. -
Without the baseline clock for clock comparison (4.5 sandy vs 4.5 ivy), i'm afraid these results are pretty much useless. It's like doing an uncontrolled experiment and passing it off as real science.Reply
-
digiex as the manufacturing process gets smaller = Smaller die size, supposed to be cooler temperature,Reply
but, with small die size = small area for heat dissipation,
...an irony that needs to be solved. -
slicedtoad Recommending a closed loop liquid cooler? really?Reply
They perform worse than decent air coolers. The lower end ones (think corsair's h60) perform like mid-low range air coolers and cost more. The better ones (h100 or antec's 920) perform on par or worse and with more noise than a similarly priced noctua. If noctuas looks too ugly for you, phantek and several others offer similar performing models.
The only reason to get closed loop lc is for looks. I admit they do give your build a nice clean look. That doesn't warrant "So, we're recommending a closed-loop liquid cooling setup, at least" though. If you'd changed that to "We're recommending higher end aftermarket coolers for a decent oc", it would've made more sense.
Anyway, I'm just nit-picking a single line from the article. All in all, it was a good read. It just makes me upset to hear wrong advice. -
_Pez_ there is no performance advantage over the i7 2600k except for power consumption. I better buy an i7-3930k.Reply -
mesab66 Absolutely true, digiex.......not far off the animal world parallel - check out the different temperature regulation methods here between large and small animals, particularly how difficult it is to regulate as the size becomes smaller.Reply -
I would like claims that Ivy Bridge procesor is overheating considerably more then Sandy Bridge addressed. And I thin k Intel really should make a better overall product and not cheat us like this.Reply